Invited
Speaker
Programmable Pro-Prodrug Systems
Rafik Karaman
Recently we have been engaged in (a)
developing a pro-drug entity to be exploited as a host for different
classes of amine drugs that upon their penetration to the human body
can release the active drug in programmable manner (Chart 1). (b)
Developing candidates for potent anti-Parkinson pro-prodrugs that
might possess a relatively high bioavailability and can enter the
blood brain barrier upon administration to the human body by a variety
of different dosage forms (Chart 2).
For achieving the goal in (a) we have chosen to exploit the trimethyl
lock system (Cohen’s model).1 Our interest in examining this
model stems from the need to make a chemical device that is composed
of a drug and an entity that binds to the drug and can undergo a rapid
reaction upon administration to the human body to furnish the drug
and the pharmacologically inactive moiety. This device is known as
a chemically driven pro-prodrug (Chart 1a). There is a pressing need
for such devices since a significant number of drugs have low solubility
in water so that their use in intravenous injection (I.V.) dosage
forms is not feasible. Linking these drugs to an entity such as hydroxyhydrocinnamic
acid system enables them to be used intravenously due to the higher
water solubility of the drug-hydroxyhydrocinnamic acid complex (pro-prodrug).
Furthermore, this system can be utilized as a programmable chemical
device that can release the active drug in a rate that is controlled
by the nature of the groups on the phenyl ring.
In the past ten years some prodrugs based on hydroxyhydrocinnamic
acid derivatives have been introduced. 2 For example, Borchardt et
al reported the use of the 3-(2’-acetoxy-4’, 6’-dimethyl)-phenyl-3,
3-dimethylpropionamide derivative (pro-prodrug) that is capable of
releasing the biologically active amine (drug) upon acetate hydrolysis
by enzyme triggering (Chart 1b). Another successful example of the
pharmaceutical applications for examining Cohen’s model is the
prodrug Taxol which enables the drug to be water soluble and thus
to be administered to the human body via intra-venous (I.V.) injection.
Taxol is the brand name for paclitaxel, a natural diterpene, approved
in the U.S.A for use as anti-cancer agent.
For fulfilling the goal in (b), Menger’s tri-carboxylic acid
shown in Chart 2 was chosen as a host for dopamine.3 This chemical
device is capable of being a new pro-prodrug for the treatment of
Parkinson’s disease that posses the following characteristics:
(1) a relatively, high bioavailability due to the capability of the
pro-drug to penetrate the blood brain barrier, (2) a moderate Hydrophilic
Lipophylic Balance (HLB) for achieving two goals: (i) maximum absorption
once the pro-prodrug enters the body tissues, and (ii) to enable the
use of the drug in different dosage forms; (3) a controlled cleavage
of the pro-drug moiety to dopamine and to a non-toxic inactive host.
Controlling the pro-prodrug cleavage rate provides a chemically driven
controlled release system that librates dopamine once the pro-drug
reaches the human brain system.
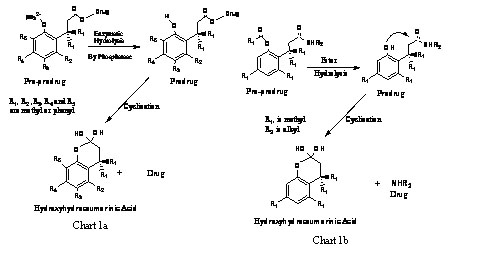
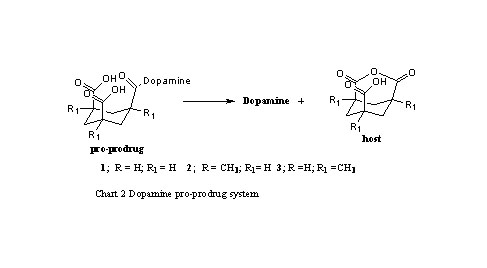
For fulfilling the two goals in (a) and (b) we sought to theoretically
investigate the driving force(s) for the significant enhancements
in rate of some intramolecular processes that have been utilized as
enzyme models and pro prodrug hosts. Using molecular mechanics, DFT
and ab initio levels of theory, we investigated the thermodynamic
and kinetic properties of a) acid-catalyzed lactonization of hydroxy-acids
as studied by Cohen 1 and Menger 3 and b) intramolecular proton-transfer
in rigid systems as studied by Menger.3 The conclusions emerged from
these studies are as follows: (1) both factors, ground state strain
and proximity orientation of the two reactive centers are important
in accelerating the rate of an intramolecular process, depending on
the nature of the system. (2) The distance between the two reactive
centers in an intramolecular reaction is a crucial factor in determining
whether the reaction is inter- or intramolecular. (3) Enthalpic as
well as entropic effects are both important factors in enhancing the
rate of intramolecular process.4
References
1. S. Milstein, L. A. Cohen, Proc. Natl. Acad. Sci. U. S. A.
67 (1970), 1143. S. Milstein, L. A. Cohen, J. Am. Chem. Soc.
94 (1972), 9158.
2. For recent reviews, see: (a) D. Shan, M. G. Nicholaou, R. D Borchardt,
B. J. Wang, J. Pharm. Sci. 86 (1997) 765. (b) B. Testa, J.
M. Mayer, Drug Metab. Rev. 30 (1998) 787. (c) W. Wang, J.
Jiang, C. E. Ballard, B. Wang, Curr. Pharm. Des. 5 (1999)
265.
3. F. M. Menger, M. Ladika, J. Am Chem. Soc. 110 (1988),
6794. F. M. Menger, Acc. Chem. Res. 18 (1985), 128. F. M.
Menger, Tetrahedron 39 (1983), 1013. F. M. Menger, Pure
Appl. Chem. 77 (2005), 1873 and references therein.
4. R. Karaman, Tet. Lett. 49 (2008), 5998. R. Karaman, Bioorg.
Chem. 37 (2009), 11. R. Karaman, Tet. Lett. 50 (2009),
452. R. Karaman, Res. Lett. Org. Chem. doi: 10.1155/2009/240253.
R. Karaman, Bioorg. Chem. 37 (2009), 106. R. Karaman, J.
Mol. Struct. 910 (2009), 27. R. Karaman, Tet. Lett.
50 (2009), 6083. R. Karaman, J. Mol. Struct. (Theochem),
accepted. R. Karaman, Tet. Lett., accepted.
|